Renowned Speakers
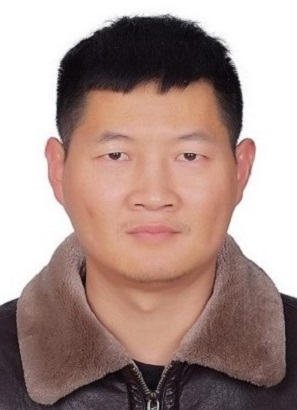
Zhaoyu Wei
Plenary Speaker, Shanghai Jiao Tong University China
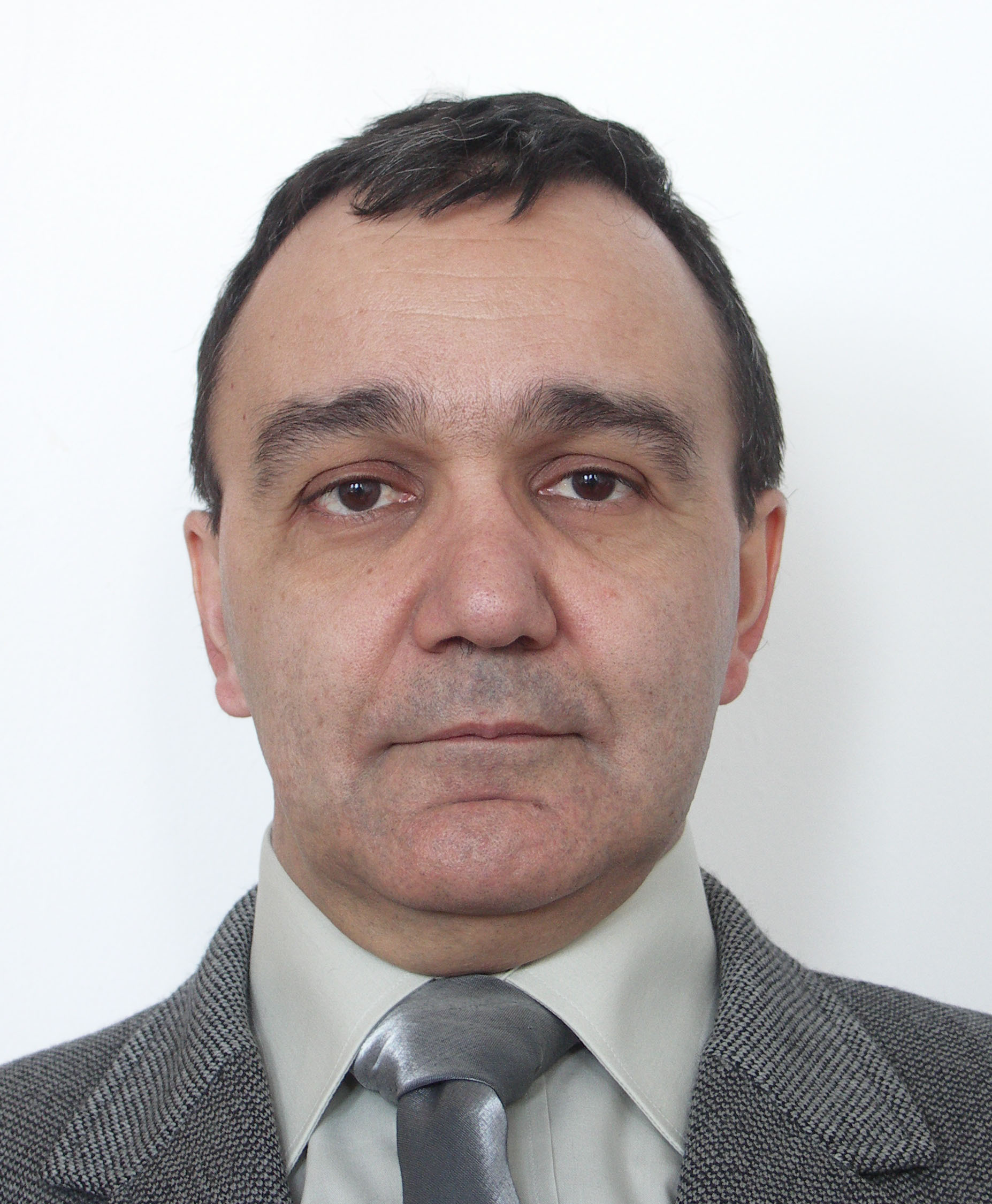
Piticescu Radu-Robert
National R&D Institute for Nonferrous and Rare Metals-IMNR, Romania
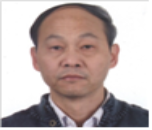
Professor Lei Yan
Key Laboratory of Space Information Integration and 3s Application, Peking University China
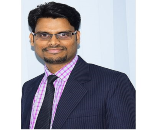
S. Anand Kumar
Additive Manufacturing Research Laboratory, Indian Institute of Technology Jammu India
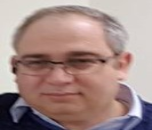
Asher Yahalom
Ariel University Israel
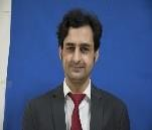
Raees Fida Swati
Institute of Space Technology, Pakistan
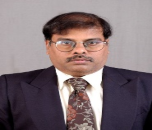
Ramprasad Srinivasan
Alliance University, India
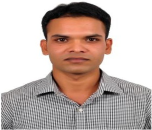
Lakshya Kumar
CSIR-National Aerospace Laboratories, India
Recommended Global EEE & Engineering Webinars & Conferences
Europe & UK
Asia Pacific & Middle East
Canada
Aerospace Conference 2022
About Conference
Aerospace Conference 2022
The organizer of the Aerospace Engineering Conference 2022 invites all participants from all over the world to join the famous scientific “2nd World Conference on Aerospace Engineering" which will take place as a Webinar on August 18-19, 2022. It is one of the world's most prestigious scientific conference like hosting scientific sessions and sub-sessions on cutting-edge research and the most recent innovations in the fields of Aerospace Engineering from all over the world. Attendees will get access to certain unique workshops and panel discussions on the most recent developments in Aerospace Engineering.
Theme: Enlighten the Future Scope development of Aerospace Engineering
Details of Aerospace Conference 2022 |
||
Conference Name |
Platform |
Date |
Aerospace 2022 |
Zoom [Webinar] |
August 18-19, 2022 |
Importance & Scope:
This is your finest opportunity to access the largest assemblage of participants from universities, colleges, research Centre’s, societies, organizations, labs, groups, communities, and enterprises etc., with members from all over the world focused on Aerospace Engineering. We wish to organize a global meeting where researchers from various controls may successfully trade data. The purpose of bringing the general public to the meetings is to catalyze empowering trades and connections between professionals in various sectors, ranging from physical science to engineering. It will create new multidisciplinary systems and allow participants to share their knowledge and data in order to achieve faster and better results.
Aerospace Engineering has not only aided in the advancement of several disciplines of research and technology, but has also contributed to the betterment of human life quality. The main goal of the Aerospace Conference 2022 is to allow attendees to connect, interact, and exchange creative ideas in diverse areas of Aerospace Engineering and Space science. The pleasure of attending Aerospace 2022 entails progressive improvement and growth in your way to doing things, as well as a broader perspective on things in international diversity.
Why to attend Aerospace 2022?
Aerospace 2022 provides a platform for globalizing research by facilitating dialogue between companies and academic institutions, as well as information transmission from research to industry. Aerospace 2022 intends to disseminate knowledge and new ideas among experts, industrialists, and students working in the fields of Aerospace Engineering by allowing them to share their research experiences and participate in interactive discussions and special sessions.
Sessions and Tracks
Track 01: Aerospace Engineering
Aerospace Engineering is the branch of engineering that deals with the design and development of Airplane and Spacecraft. Aeronautical Engineering and Astronautical Engineering are two important fields that overlap. Avionics Engineering is related to aerospace engineering, except it focuses on the electronics side of things. It covers the science and technology of Airplanes and spacecrafts, as well as their research, design, development, construction, testing, and collaboration procedures. It entails the investigation of the science and engineering that underpins the physical qualities of rockets, planes, and spacecraft. This is a complicated subject that necessitates the use of advanced and sophisticated technologies. Aerospace Engineers also work on new technology development in aviation, space exploration, and defence systems. Aerospace Engineers work on everything from planes to space shuttles to satellites to missiles. They require sophisticated math and physics skills. One of the most difficult technical fields is Aerospace Engineering. Engineers must test aircraft for safety in addition to developing and engineering them.
There are two key fields in Aerospace Engineering:
• Aeronautical Engineering: This field deals with aero planes that fly through the Earth's atmosphere.
• Astronautical Engineering: This field deals with spacecraft that operate outside of Earth's atmosphere.
Roles of Aerospace Engineer:
• An Aerospace Engineer is in charge of directing and organizing the design of aircrafts and aerospace products, as well as producing and testing them.
• Aerospace Engineers make sure that the project on which they are working shall result in safe aircrafts and their parts.
• Aerospace Engineers check faulty or damaged items in order to identify the sources of faults and recommend feasible fixes.
• Aerospace engineers are in charge of overseeing the building of aircraft, as well as the installation of engines, instruments, and other equipment.
• They must take part in flight test programmers to determine rate of ascent, stall speeds, take-off lengths, and landing capabilities.
Track 02: Aerodynamics
Aerodynamics is a discipline of dynamics concerned with the motion of air and other gaseous fluids, as well as the forces acting on moving bodies in relation to these fluids. Air circulates around objects in this manner. The laws of aerodynamics describe how an airplane can fly. Aerodynamics affects anything that travels through the air. It affects a rocket blasting off the launch pad and a kite flying into the sky. It also affects automobiles, as air flows around them. In aerodynamics, there are three primary forces to consider: thrust, which propels an airplane forward, drag, which holds it back, and lift, which keeps it aloft. Cars, bicycle racing helmets, wind turbines, and golf balls are examples of technologies that rely on aerodynamics. Aerodynamics is the science of how air travels around objects. There are many examples of aerodynamic technology outside of aircraft because air is all around us. The study of how gases interact with moving bodies is known as aerodynamics. Aerodynamics is largely concerned with the forces of drag and lift induced by air flowing over and around solid bodies, because air is the most common gas that humans encounter. For example, in order for a plane to stay in the air, the lift force must be greater than the gravitational force. The push must also overcome the drag force, which opposes the plane's passage through the air. A flow is said to be compressible if its density changes along a streamline, according to aerodynamic theory. This means that, unlike incompressible flow, density variations are taken into account. In general, this is the situation, when the Mach number in part or all of the flow reaches 0.3. The top of an airplane's wing is curved, whereas the bottom is flat. Because of that, air flows faster over the top than under the bottom. As a result, there is less air pressure on top of the wing. The wing, as well as the airplane to which it is attached, moves up as a result of this circumstance. Airplanes are able to fly because they can generate a force known as lift, which propels the plane upward. The forward motion of the airplane through the air generates lift. The engine's thrust is responsible for this motion. The field of applied aerodynamics encompasses a wide range of applications, including fixed-wing and rotary-wing aircraft, wind turbines and propellers, ground and sea vehicles, internal flows, bird and insect flight, and atmospheric flows.
Track 03: Aircraft Design and Structures
The aircraft design process is a loosely defined approach for balancing multiple conflicting and demanding objectives in order to generate a sturdy, lightweight, and cost-effective aircraft. It can carry a significant payload while remaining reliable enough to fly safely for the duration of the aircraft's design life. Airplanes and other types of aircraft, such as helicopters, are designed by Aeronautical Engineers. They concentrate on the unique aspects of flight within the Earth's atmosphere. To meet customer expectations, ensure quality standards, meet FAA rules, and keep to budget and project dates, design engineers will construct complicated designs of aircraft interiors, principal or secondary structures or mechanical systems. One of the two fields of aerospace engineering is aeronautical engineering. There are three primary phases to the aircraft design process. Conceptual Design, Preliminary Design, and Detail Design are the three phases. Each phase focuses on a different topic. Aerospace engineers work in industries that design or produce aircraft, missiles, national security systems, or spacecraft. Manufacturing, analysis and design, research and development, and the federal government employ the majority of aerospace engineers. The fuselage, wings, empennage, undercarriage, and one or more engines are all major components of an airplane. Wind tunnels are used by researchers to understand more about how an aircraft will fly. Wind tunnels are used by NASA to test scale models of planes and spacecraft. Some wind tunnels are large enough to accommodate full-size cars. The wind tunnel creates the illusion that an object is flying by moving air around it. A fuselage, wings, empennage, landing gear, and a power plant are all common airplane structures. The fuselage is the plane's main body, and it's meant to hold the crew, passengers, and cargo. It also acts as a structural link between the wings and tail assembly. Aircraft structures are made of aluminum, titanium, and alloy. Titanium is employed in the manufacture of numerous aircraft parts as well as engines. Titanium is also used for the wings and landing gear. The Fuselage, Wings, Empennage, Power Plant, and Landing Gear are the five main components of an aircraft. The primary structure is the one that carries flight, ground, or pressurization loads and whose failure would jeopardize the airplane's structural integrity. Secondary structures are those that are not primary load-bearing components and whose breakdown would not compromise the airframe's structural integrity or prevent the plane from flying and landing safely. The Small Airplane Directorate released AC 23-19, which uses the same terminology.
Comparing the different types of Aircraft Fuselage Structures:
Truss: A truss structure fuselage is typically built of welded steel tube trusses and is commonly utilized in lightweight aircraft (though it can also be made of wood). To obtain a more aerodynamic design, they are frequently rounded and have lightweight stringers.
Geodesic: Geodesic fuselage structures aim to improve the shape of the plane in order to reduce drag and increase speed. Similar to a wicker basket, many strip stringers are wrapped around the formers in varied spiral directions. Geodesic fuselage structures are light, sturdy, and extremely long-lasting. They were usually made of wood or metal and had a cloth covering over the shell for added comfort and aesthetic appeal.
Monocoqne: The fuselage is designed within the aircraft's principal structure in the monocoqne shell construction. It was made out of moulded plywood with various layers that cover a mould plug. A fiberglass-type fabric with polyester resin, as well as a derivative of similar design with epoxy resin, is other forms of the monocoqne shell.
Semi-Monocoqne: When building an all-aluminum fuselage, the semi-monocoqne fuselage structure is preferred. It has frames that form the fuselage's shell and are held in place by cross sections on a rigid fastening. Stringers are used to connect the fixture to the stringers. These are only a few of the most popular fuselage structures in aviation. All planes, from small single-engine planes to bigger commercial jets, require a fuselage. It's a necessary component for efforts to convey people and freight.
Track 04: Aerothermodynamics and Propulsion
Aerothermodynamics is the precise and conservative forecast of the heating environment that an earth or planetary entry vehicle will encounter.
• The TPS is designed to survive the projected environment with risk acceptable margins.
• Aero thermal modeling is connected and interwoven with Thermal Protection System (TPS) design.
• In ablative systems, the flow field and TPS interact in a non-reversible way; the physics are coupled.
This area of study encompasses launcher and spaceship propulsion systems, as well as atmospheric ascent and re-entry. Chemical, electric, and sophisticated systems are all examples of propulsion technologies used to propel rockets and spacecraft. Aerothermodynamics is the study of how high-velocity gases behave, which is used to improve engine design and mimic the process of ascent and re-entry into the atmosphere. The fields of propulsion and aerothermodynamics are critical for maintaining and expanding our space gateway. To get a mission to where it needs to be, it involves great speed and precisely regulated thrust shooting. Landing on earth or other planets is equally difficult, especially because the high velocity that sent the spacecraft into space must be bled away to enable a safe landing. Chemical propulsion, which uses liquid propellants that are kept in a cooled liquid condition aboard the rocket or spacecraft fuel tank, remains state-of-the-art for the large thrust provided by current launch vehicles as well as on-orbit spacecraft operations. Solid rocket motors can be employed for 'kick' stages that place payloads into final orbits, while cold gas systems, which merely expel pressurized gas through a nozzle, are best suited to small spacecraft or low thrust applications. Electric propulsion systems, which use electrical energy to propel their fuel to high speeds, are becoming increasingly important in spaceflight. Electrostatic engines, in particular, are appreciated for their capacity to provide continuous thrust over long periods of time while using little amounts of fuel.
Track 05: Engineering Mathematics
Engineering mathematics is an area of applied mathematics that deals with mathematical concepts and procedures utilized in engineering and industry. Engineering mathematics, like engineering physics and engineering geology, is an interdisciplinary discipline inspired by engineers' needs for practical, theoretical, and other concerns outside of their specialty, as well as to deal with limits in order to be effective in their work. Engineering mathematics is the art of using math to solve complicated real-world issues by combining mathematical theory, practical engineering, and scientific computers to solve today's technology problems. Mathematicians tackle economic, scientific, technical, and business problems using mathematical theory, computational approaches, algorithms, and cutting-edge computer technology.
Track 06: Flight Mechanics
Lift, thrust, drag, and weight are the four forces in flight mechanics. Lift keeps a Frisbee afloat as it flies through the air. Lift is an upward-acting force; drag is a retarding force caused by the aircraft's resistance to lift and friction as it moves through the air; weight is the downward influence of gravity on the aircraft; and thrust is the forward-acting force generated by the propulsion system. Fixed wing (gliders, airplanes) and rotary wing (helicopters) aircraft are both affected. In ICAO Document 9110, an airplane is defined as "a power-driven heavier than air aircraft, deriving its lift largely from aerodynamic reactions on surface that remain fixed under specific flying conditions."
Take-off: Only a succession of aerodynamic forces can propel a heavier-than-air craft (plane) into the air. The fuselage of a fixed-wing aircraft holds up the wings before takeoff in fixed-wing aircraft. The reverse occurs at takeoff, with the wings supporting the plane in flight.
Straight and level flight of aircraft: A powered aircraft is influenced by four forces while in flight: lift, weight, thrust, and drag. Thrust is the force generated by an engine (whether it's a jet engine, a propeller, or — in unusual situations like the X-15 — a rocket) that operates in a forward direction to overcome drag. The vector describing the aircraft's velocity in relation to the atmosphere is perpendicular to lift. Drag acts in the opposite direction of the aircraft's velocity vector because drag resists motion through the air. The weight of the airplane is transferred to the Earth's center of gravity through the aircraft's center of gravity.
Aircraft control and movement: An airplane can shift its attitude in relation to the moving air in one of three ways. Pitch (movement of the nose up or down, rotation around the transversal axis), roll (rotation around the longitudinal axis, that is, the axis that spans the length of the aircraft), and yaw (movement of the tail up or down, rotation around the longitudinal axis) are the three types of rotation (movement of the nose to left or right, rotation about the vertical axis). The aircraft must first roll to achieve an angle of bank (in order to produce a centripetal force) before turning (change of heading). Once the required change of heading has been achieved, the aircraft must be rolled in the opposite direction to lower the angle of bank to zero. Lift is generated vertically through the center of pressure, which is determined by the position of the wings. The position of the center of pressure will fluctuate when the angle of attack and the setting of the aircraft wing flaps alter.
Track 07: Fluid Mechanics
The study of fluid behavior (liquids, gases, blood, and plasmas) at rest and in motion is known as fluid mechanics. It has numerous applications in mechanical and chemical engineering, as well as biological and astrophysical systems. The continuity equation (i.e. mass conservation), the momentum principle (or momentum conservation), and the energy equation are the three basic fluid mechanics principles. The Bernoulli equation, which is derived from the motion equation, is a related principle. Fluid mechanics is divided into two branches: fluid statics, which is the study of fluid in a stationary condition, and fluid dynamics, which is the study of fluid in motion. Fluid Flow is a branch of fluid mechanics that studies fluid dynamics. The motion of a fluid subjected to uneven forces is involved. As long as unbalanced pressures are applied, this motion will persist.
Types of Fluids:
Ideal fluid: When a fluid cannot be compressed and its viscosity does not fall into the category of an ideal fluid, it is considered to be ideal. It's a made-up fluid that doesn't exist in real life.
Real fluid: All fluids are real since they all have viscosity.
Newtonian fluid: It is defined as one that obeys Newton's law of viscosity.
Non-Newtonian fluid: It is defined as a fluid that does not obey Newton's law of viscosity.
Ideal plastic fluid: It occurs when shear stress is proportional to velocity gradient and shear stress exceeds the yield value.
Incompressible fluid: It is one in which the density of the fluid does not change when an external force is applied.
Compressible fluid: It occurs when the density of a fluid changes when an external force is applied.
Track 08: Thermodynamics and Propulsion
The study of the relationships between heat, work, temperature, and energy is known as thermodynamics. The rules of thermodynamics define how energy evolves in a system and whether it can do beneficial work on its surroundings. Heat is a type of energy that corresponds to a specific quantity of mechanical work, which is the central notion.
The most important laws of thermodynamics are:
•The zeroth law of thermodynamics: In which states that the first two systems are in thermal equilibrium with each other when they are each in thermal equilibrium with a third system? This trait makes using thermometers as a "third system" and defining a temperature scale meaningful.
•The first law of thermodynamics: It also known as the law of energy conservation. The difference between heats added to the system from its surroundings and work done by the system on its surroundings equals the change in a system's internal energy.
•Thermodynamics' second law: Heat does not spontaneously flow from a colder to a hotter region, or, to put it another way, heat at a particular temperature cannot be turned fully into work. As a result, a closed system's entropy, or heat energy per unit temperature, rises over time to a maximum value. As a result, all closed systems converge to a state of equilibrium in which entropy is greatest and no energy is available to conduct beneficial work.
•Thermodynamics' third law: As the temperature approaches absolute zero, the entropy of a perfect crystal of an element in its most stable form decreases. This enables the creation of an absolute entropy scale that determines the degree of entropy from a statistical standpoint. This enables the creation of an absolute entropy scale that, from a statistical standpoint, determines the degree of randomness or disorder in a system.
Propulsion often refers to the engine technology that provides the energy required lifting an airplane from the end of a runway into the air and keeping it there and most people think of jet engines or single or dual propeller engines seen on large and small passenger planes. The force of being driven, or a device that creates movement, is known as propulsion. The propelling force of a paper airplane is an example of propulsion. A propulsion system is a machine that generates thrust in order to propel a vehicle ahead. Thrust is frequently generated on airplanes by employing Newton's third law of action and reaction. Second, the thrust from the propulsion system must be greater than the airplane's drag in order for it to accelerate. The propulsion system's purpose is to generate thrust. The power that propels a rocket through the air and into space is called thrust. Thrust is produced by the rocket's propulsion system. The power of the propeller machinery, measured at the coupling flange of the shaft and represented in kilowatts, is referred to as propulsion power. Within aerospace engineering, propulsion engineering is a specialty.
Engineers who work in propulsion design, the engines that allow rockets, missiles, satellites, and other spacecraft to accelerate for launch or flight in space.
Depending on the type of propulsion used in an aircraft, thrust is created in a variety of ways:
• Turbojet: The thrust is created entirely by jet outflow from the engine's back. (Now primarily utilised in military aircraft)
• Turbofan: a big fan at the front of the engine generates the majority of the thrust; a small percentage is provided by jet outflow.
• Turboprop: The propeller generates the majority of thrust, with only a minor percentage coming from jet exhaust.
• Piston: the propeller generates all of the thrust.
Track 09: Turbomachinery
In mechanical engineering, turbomachinery refers to machines that transmit energy between a rotor and a fluid, such as turbines and compressors. A compressor transfers energy from a rotor to a fluid, whereas a turbine transfers energy from a fluid to a rotor. TURBOMACHINERY ENGINEER, AERODYNAMICS AND HYDRODYNAMICS leads SpaceX's design, development, and validation of rotating and stationary flow path components for a variety of turbomachinery applications, from idea to flight or other operations. There are two types of turbo machines: those that absorb power to increase fluid pressure or head (ducted and unducted fans, compressors, and pumps) and those that produce power by expanding fluid to a lower pressure or head (ducted and unducted fans, compressors, and pumps) (wind, hydraulic, steam, and gas turbines).
The main components of a turbo machine are as follows: The three components are the rotor, the stator, and the shaft. The rotor blades or vanes are carried by a spinning unit called a rotor. Runners, impellers, and other terms for rotors are used depending on the machine. A rotorcraft, often known as a rotary-wing aircraft, is a heavier-than-air aircraft with rotor blades that rotate around a vertical mast to generate lift. A rotor is a collection of rotor blades set on a single mast. The stationary airfoils, also known as vanes or stators, transform the additional kinetic energy into static pressure and reverse the fluid flow direction to prepare it for the following stage's rotor blades. The shaft runs the length of the engine, connecting the turbine to the compressor. There could be three concentric shafts spinning at different speeds, each with its own set of turbines and compressors. The compressor may supply cooling air to the turbines through the shaft.
A portion of turbo machinery is required in almost every sort of renewable energy cycle. Compressors, fans, blowers, pumps, and turbines are all essential components in a variety of renewable energy applications, ranging from wind to ocean energy, bottoming cycles, and hydrogen pumping. A turbo machine that absorbs power turns mechanical energy into fluid energy. The best examples of this category are compressors, fans, pumps, and blowers. The term "power transmitting" refers to a system that uses fluid to transfer power from the driving shaft to the driven shaft.
Track 10: Aviation Communication
Aviation communication is the process by which aircraft operators communicate with other planes and persons on the ground. Aviation communication is a critical component for the safe and efficient operation of aircraft on the ground and in the air. Communication is critical in aviation since it helps to boost safety and reduce incidents that may have been avoided. Very high frequency (VHF) radio calls are the most common mode of communication in aviation, accounting for over 95 percent of our conversations with ATC. In simple terms, the transmitting station sends a signal that is picked up by the receiving station and travels in a straight line. Verbal, nonverbal, written, written and graphical, as well as human-machine and machine-machine communications, are the five types of aviation communication. The most prevalent mode of communication in an aircraft or in the aviation sector is verbal communication, sometimes known as speech. An aviation maintenance worker will communicate in a variety of ways during the day. Oral and written communications are the two most prevalent techniques to deliver a message. Some people are stronger at verbal communication but need to work on their written communication, and vice versa. Radio waves are utilized for communication, nautical and aviation navigation, and wireless transfer of sound messages or information. A pilot signal is a signal delivered through a communications system for supervisory, control, equalization, and continuity, synchronization, or reference purposes, usually at a single frequency. ACARS messages can be classified into three categories based on their content: ATC (Air Traffic Control), AOC (Aeronautical Operational Control), AAC (Airline Administrative Control).
Track 11: Applications of Aerospace Technology
The construction, testing, and maintenance of airplanes and space vehicles are referred to as aerospace technology. Technicians may work on systems linked with dependable and reusable space launch vehicles and related ground support equipment, including assembly, servicing, testing, operation, and repair. Aeronautical engineering, satellite communications, guidance and tracking systems, destructive and non-destructive materials testing, propulsion and engine testing, surveillance and electronic warfare, impact studies, mechanical testing, and vibration analysis are just a few examples of typical aerospace applications. Augmented and virtual reality (AR/VR), the Internet of Things (IoT), Artificial Intelligence, and 3D printing are all influencing different aspects of aeronautical engineering, including avionics. Aluminum alloys, titanium alloys, high-strength steels, and composites are the most regularly utilized commercial aerospace structural materials, accounting for more than 90% of the weight of airframes.
Aerospace Applications:
•Radiation monitoring and dosimetry.
•Radiation-hardened components.
•Superconducting and cryogenic systems.
•Thermal management, advanced materials and processes.
•Big data handling and analysis tools.
•Irradiation and cryogenic testing facilities.
6 Technologies:
•Airline New Distribution Capability
•Indoor positioning systems (Beacons technology)
Track 12: Spacecraft and Space Engineering
A spacecraft is a vehicle or machine that has been developed to go through space. Spacecraft are a form of artificial satellite used for a variety of functions, including communications, Earth observation, meteorology, navigation, space colonization, and planetary exploration, and person and freight transportation. A spacecraft's launch is defined as a period of powered flight in which the vehicle climbs beyond Earth's atmosphere and accelerates to orbital velocity. When the last stage of the rocket burns out, the spacecraft separates and continues to fall in free-fall. Aluminum is the material of choice for spacecraft construction since it is both robust and light. However, metal isn't very effective at blocking radiation. Plastic, on the other hand, appears to be a more superior material. The most basic spacecraft control themselves by spinning or reacting with the Earth's magnetic or gravity fields. They might be uncontrollable at times. Spacecraft may have several bodies or be linked to essential sections that require individual attitude pointing, such as solar arrays or communication antennas. A spaceship structure's different design aspects and phases (spacecraft configuration, initial design, comprehensive analysis, structure manufacture, and testing). Spaceship Engineer is a term used to describe a spacecraft designer.
Space Systems Engineering is a programmer in aerospace engineering that concentrates only on astronautical engineering, or the design of spacecraft and space systems. Aeronautical engineering (plane) and astronautical engineering (spacecraft) are two types of aerospace engineering (spacecraft and launch vehicles). Engineers in the space industry create, build, and maintain spacecraft and systems. They are in charge of launching spacecraft, landing rovers on Mars, and developing communication satellites that enable us to utilize the Internet, among other things. Aeronautical engineering is a branch of engineering that deals with the design, development, construction, testing, and operation of vehicles that operate in the Earth's atmosphere or in space.
Track 13: Space Dynamics
Space dynamics is the study of bodies in motion in space. Celestial, Mechanics, and Space Dynamics are all terms used to describe the study of space dynamics. The study of the motion of man-made objects in space as they are subjected to natural and manmade influences. The Space Dynamics Laboratory (SDL) provides sensor systems and supporting technologies to the US government to address challenges. SDL creates real-time data reconnaissance systems, designs and develops electro-optical sensors, constructs tiny satellites, provides calibration services, and designs electro-optical sensors. The world's space activities are one of humanity's most significant achievements. It enables real-time communications, study of Earth resources, weather forecasting, precise positioning, and a variety of other tasks that are already commonplace in our life. The study of space dynamics is critical in these breakthroughs because it helps us to plan how to launch and operate a space vehicle to achieve the desired objectives. The study of Celestial Mechanics and Control applied to spacecraft and natural objects is included in this field. The main objectives are to determine the orbit and attitude of the spacecraft based on observations, to obtain its position and attitude in space in a given time from some initial conditions, to determine the best way to change their orbits and attitudes, and to analyze how to use satellite information to find the position and velocity of a given point (e.g., a personal receptor, a satellite or a car).
Track 14: Wind Tunnel Testing
Wind tunnels are giant tubes with air rushing through them that are used to simulate the interaction of air with an object that is flying through the air or moving along the ground. Wind tunnels are used by aerodynamicists to test models of prospective aircraft and engine components. The model is put in the test part of the tunnel and air is forced past it during the test. The forces on the model are measured using a variety of instruments. NASA uses wind tunnels to evaluate ideas for improving and securing airplanes. Engineers can put innovative materials or shapes for plane parts to the test. Then, before flying a new plane, NASA will put it through a wind tunnel test to ensure that it will fly properly. The air in a wind tunnel is normally moved through the tube by strong fans. The thing to be tested is placed in the tunnel in such a way that it cannot move. The air moving around the static object represents what would happen if the object were in motion. The settling chamber, the contraction cone, the test bed, the diffuser, and the fan housing with a variable speed motor are the five essential components of the wind tunnel, ordered from front to back. In order to provide very accurate results in drag measurement, transonic wind tunnel testing must produce results within 1 count (1 count = around 0.3 percent of total resistance, which is comparable to 1–2 seats). Wind tunnels are built with a certain function in mind and for a specified speed range. A series of spinning vanes convey air from the exit of the test section back to the fan in the closed return tunnel. The air is returned to the contraction portion and back via the test section after exiting the fan. The model is instrumented in certain wind tunnel tests to offer diagnostic information about the flow of air around the model. Static pressure taps, tufts on the surface, and total pressure rakes are examples of model instrumentation. Models of wind tunnels are complicated and expensive pieces of machinery. Wind tunnels are commonly used in aerodynamic research to study the behavior of flows in channels and across solid surfaces under various conditions. Aerodynamicists can utilize the wind tunnel's controlled environment to measure flow conditions and forces on aircraft models as they are being developed. Low-speed and high-speed wind tunnels are divided into subsonic (80 percent of the speed of sound), transonic (about the speed of sound), supersonic (up to 6 times the speed of sound), hypersonic (6 to 12 times the speed of sound), and hypervelocity (up to 12 times the speed of sound).
Track 15: Advanced Tactical Airborne Reconnaissance System (ATARS)
The system that is commonly used for image collection, data storage, and data linking. IR and visible sensors, digital recorders, a Reconnaissance Management System, an interface with Radar that records synthetic aperture radar pictures and a digital data link were all part of the Advanced Tactical Airborne Reconnaissance System (ATARS) of some advanced aircraft. This system includes a small data link pod that may transmit data (imagery and auxiliary) to any Imaging Station (CIG/SS in common) that is compatible. The United States Marine Corps uses the Advanced Tactical Airborne Reconnaissance System (ATARS) on its F/A-18D Hornet aircraft for image acquisition, data storage, and data communication. It includes infrared and visible light sensors, two digital tape recorders, and a Reconnaissance Management System (RMS); an interface with the APG-73 Radar Upgrade (Phase II) that records synthetic aperture radar (SAR) imagery; and a digital data link mounted in a centerline pod. The nose gun is replaced with ATARS, which has a tiny data link pod positioned on the centerline station. The digital data link will send imagery and auxiliary data to any CIG/SS compatible system, such as the Joint Services Imagery Processing System (JSIPS) or Marine Tactical Exploitation Group (TEG) situated ashore and Navy JSIPS (JSIPS-N) onboard ship. Three ATARS aircraft are assigned to each of the four US Marine Corps F/A-18D squadrons, for a total of 12 ATARS aircraft. In February 2000, MCAS Beaufort-based VMFA(AW)-332 deployed to Hungary in Operation Allied Force, marking the first operational usage of ATARS equipped aircraft. ATARS is a significant improvement over the Marines' outdated RF-4B/C aircraft in terms of capability.
Track 16: Intelligence, Surveillance, Target Acquisition and Reconnaissance (ISTAR)
ISTAR stands for intelligence, surveillance, target acquisition, and reconnaissance. ISTAR is a practice that brings together multiple battlefield operations to help a combat unit better use its sensors and manages the data they collect. On the battlefield, data is gathered through methodical observation by deployed soldiers as well as a variety of electronic devices. This information can be obtained by surveillance, target acquisition, and reconnaissance. The information is subsequently passed on to intelligence professionals for processing, and finally to the commander and his staff for battle plan creation. Intelligence is information that has been processed and adds to a better knowledge of the situation on the ground, as well as enemy dispositions and intentions. ISTAR is the process of combining intelligence with surveillance, target acquisition, and reconnaissance tasks to increase a commander's situational awareness and, as a result, decision-making. The presence of the "I" is significant since it emphasizes the need of processing data from all sensors into valuable knowledge. The action of equipping armed forces with information and intelligence to help in combat roles and other operational activities is known as intelligence, surveillance, target acquisition, and reconnaissance (ISTAR). This is frequently the result of linked information networks that improve situational awareness. Raytheon Intelligence & Space's ISTAR is a multi-intelligence aircraft that provides essential information to military and civilian air and ground operators using artificial intelligence, integrated onboard sensors, and advanced processing. Visual observation (from soldiers on the ground) and electronic observation (from satellites, unmanned aircraft systems, ground sensors, and maritime vessels) are used in both surveillance and reconnaissance, which is then analyzed to turn data into intelligence.
Track 17: Robotics and Rotorcraft
Robotics is an engineering discipline that deals with the conception, design, construction, and operation of robots. The goal of robotics is to develop intelligent devices that can help people in a number of ways. Robotics can come in a variety of shapes and sizes. Robotics is the study of the design, building, and use of machines (robots) to do jobs that were previously performed by humans. Robots are commonly utilized in areas such as vehicle manufacturing to perform simple repetitive jobs, as well as in businesses where workers must work in dangerous situations.
A rotorcraft, often known as a rotary-wing aircraft, is a heavier-than-air, aircraft having rotary wings or rotor blades that rotate around a vertical mast to generate lift. A rotor is a combination of several rotor blades set on a single mast. "Supported in flight by the responses of the air on one or more rotors," according to the International Civil Aviation Organization (ICAO). Helicopters, autogyros, and gyrodynes are examples of rotorcraft. Additional propulsion engines, propellers, or static lifting surfaces are used in compound rotorcraft to supplement the rotor. The Bernoulli Principle, which applies to wings, creates lift. Because of a link known as the Bernoulli Principle, wings provide lift. The Bernoulli Principle defines the relationship between air speed and pressure. To produce lift, an airplane must fly fast enough to move enough air over its wings. By rotating its blades, a helicopter propels air over its rotor.
Track 18: Avionics and Mission Technologies
Electronic Equipment Used in the Air' is how avionics is defined. From the Engine Computers to the Fly-by-Wire Flight Control System, this simple concept affects almost every component of a modern airplane. In reality, each A380 aircraft has nearly 500 kilometers of wire. Avionics (a combination of aviation and electronics) refers to the electronic systems found aboard planes, satellites, and spacecraft. Communications, navigation, the display and administration of various systems, and the hundreds of systems added to aircraft to perform distinct functions are all examples of avionic systems. Engine controls, flight control systems, navigation, communications, flight recorders, lighting systems, threat detection, fuel systems, electro-optic (EO/IR) systems, weather radar, and performance are all examples of avionics that can be found in an aircraft or spacecraft. The avionic systems are necessary for the flight crew to safely and efficiently complete the aircraft task. Continued on the Importance and Role of Avionics Transporting passengers to their destination, intercepting a hostile aircraft, assaulting a land target, reconnaissance, or maritime patrol are all possible missions.
New Avionics Technologies:
•Advanced Wireless Flight Sensor System
•Real-Time Parameter Identification
•Mechanoluminescent Materials for Structural Health Monitoring
•ARMD Flight Data Portal (AFDP)
•Atmospheric Science Team Supports Key Flight Missions
•Ethernet via Telemetry (EVTM)
•Flight Test Instrumentation for Advanced Propulsion Systems
•Armstrong Operates and Maintains Flying Observatory
•Portable Data Acquisition System (PDAT)
•Distributed Aero structural Sensing and Control
Market Analysis
Aerospace is a large and complex multinational sector that includes fuel, deicing, airports, retail, and traffic control, as well as a military and commercial component. A typical modern aeroplane contains over three million parts from over 800 different companies in ten different countries, therefore study into this highly complex vertical necessitates a thorough grasp of the supply chain. In the engineering sector, the aircraft industry is one of the most globally integrated. In 2016, the global aerospace engineering market was worth US$ 70.0 billion, and it is predicted to grow at a CAGR of 4.1 percent from 2017 to 2025. Due to an increase in the total airline business around the world, the aerospace engineering industry has seen tremendous expansion in recent years. Numerous top aircraft manufacturers have been active in procurement of various aircraft components and services in order to meet the rising need for new aircraft to meet the expanding demand for air travel. Furthermore, advances in aerospace engineering have resulted in an increase in aircraft replacement. Various airline operators throughout the world strive to keep their fleets up to date and cutting-edge in order to maintain dominance in their respective markets. The global aerospace engineering industry was valued at US$ 78.96 billion in 2019, but it is expected to drop by about 42% in 2020 due to lower expenditures in aircraft as a result of travel restrictions. In 2020, the market is expected to reach US$ 45.40. With international travel set to resume in 2021 and sustained investments in UAVs, the market is expected to increase at a CAGR of 7.1 percent from 2020 to 2028, taking into account post-COVID-19 recovery. By 2025, the global aerospace engineering services outsourcing market is expected to reach USD 188.24 billion, representing a CAGR of 25.9% during the forecast period.
The market research on aeronautical engineering is divided into the following categories:
Aerospace engineering market by component:
• Engineering Services • Aero structures
Aerospace engineering market by application:
• Aircrafts • Spacecraft
• Regions The following topics are covered in the Aerospace Engineering Market Report:
• North America (the United States, Canada, and Mexico) • Europe (Germany, the United Kingdom, France, Italy, Russia, and Turkey, among others) • Asia-Pacific (China, Japan, Korea, India, Australia, Indonesia, Thailand, the Philippines, Malaysia, and Vietnam) (Egypt and GCC Countries)
• The key markets for Aerospace Engineering are still Europe, North America, and Asia-Pacific. Market participants are increasing their worldwide presence and product offerings in response to new opportunities. On the one hand, Aerospace Engineering producers strive to reduce their manufacturing costs; on the other hand, they strive to provide clients with higher-quality products. Furthermore, they are attempting to widen the scope of Aerospace Engineering applications.
Major Key Players Covered in the Aerospace Engineering Market Report: • WS Atkins Plc • Bombardier, Inc • Cyient Ltd • Elbit Systems Ltd • Leonardo DRS • Saab Group • Safran System Aerostructures • Sonaca Group • Strata Manufacturing PJSC • UTC Aerospace Systems • General Dynamics Corporation • Strata Manufacturing PJSC
Participation Benifits
Benefits of Participation for Speaker
- Worldwide appreciation of the profile of Researchers.
- Obtain credits for professional growth.
- Explore the latest of cutting edge analysis.
- Make long-term bonds at social and networking activities.
- An ability to advertise one page in the distribution of abstract books and flyers that ultimately gets 1 million views and adds great value to your research profile.
- Learn a transition beyond your area of interest to learn more about new subjects and studies away from your core subject of Aerospace Engineering.
- We have distinctive networking, learning and enjoyable integration into a single package.
Benefits of Participation for Delegate
- Professional Development-Improve understanding and knowledge.
- Your participation will help us with a new methodology and ideology that can be used to broaden the outcomes of businesses or industries.
- Opportunities for Aerospace Engineering Summit researchers and experts in the same field to meet and exchange new ideas.
Benefit of Participation for Sponsor
- Exposure to the international environment would increase the possibility of new companies.
- Opportunity to demonstrate your company's latest technologies, new products, or service your business to a wide range of international participants.
- Increase business by our participants through lead generation.
- It takes a lot of time, effort and drive to create a successful company, so it's always nice to have a network of colleagues and associates to draw energy from individuals who share a common drive and objective.
- Benchmarking main organization plans and moving it forward.
- Get feedback from trustworthy people at our webinar to your company questions and challenges.
- On our banner, website and other proceedings, branding and marketing content, the advertising logo of your company.
Benefit of Association for Collaborators
- Nobody has these massive visitors to Aerospace Engineering in the world; this is the best forum to highlight society.
- Creating long-lasting peer relationships.
- In our banner, website and other proceedings, branding and marketing material, promotional content and your Organization logo will increase your number of subscribers/members by 40%.
- The exposure of our event to your Company listing in the Global Business forum will have a great effect on your association.
- Your representatives can network to update their knowledge and understanding of your organisation and services with key delegates.
- Aerospace Engineering advertising materials such as posters, brochures, pamphlets, services that will be circulated to hospitals, universities, society and researchers will be integrated with information.
To Collaborate Scientific Professionals around the World
Conference Date August 18-19, 2022
For Sponsors & Exhibitors
Speaker Opportunity
Useful Links
Supported By
All accepted abstracts will be published in respective Conference Series International Journals.
Abstracts will be provided with Digital Object Identifier by